Promote your practice with ChiroHosting.
Achieve your online marketing goals with premium services designed specifically for chiropractors.
Start with the best chiropractic website.
Attracting new patients online starts with your ChiroHosting N8 Chiropractic Website.
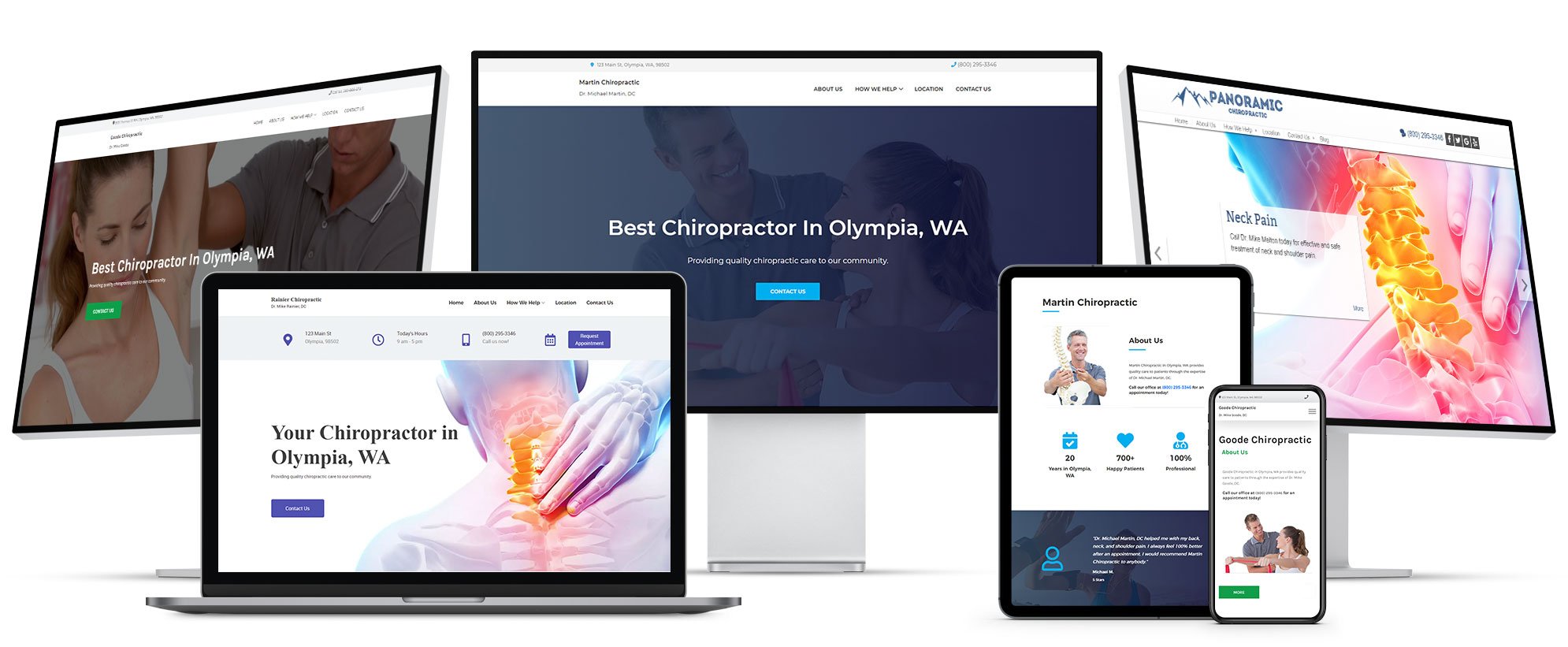
LEARN MORE ABOUT N8 CHIROPRACTIC WEBSITES
Built for your practice type.
Select your practice type and we'll build your N8 Chiropractic Website with everything you need to attract your perfect patients.
Start your website for free.
Many believe our chiropractic websites are the best available. Not only do you get more features, you get the best price.
Experience the best chiropractic website.
Now you can try out all of the features included with your N8 Chiropractic Website, for free.
- One-click theme customization
- Built-in practice type articles
- Drag-and-drop widgets
- 80+ ChiroNexus videos
- Hundreds of stock images
- Much more...
There's no obligation and no credit card is required.
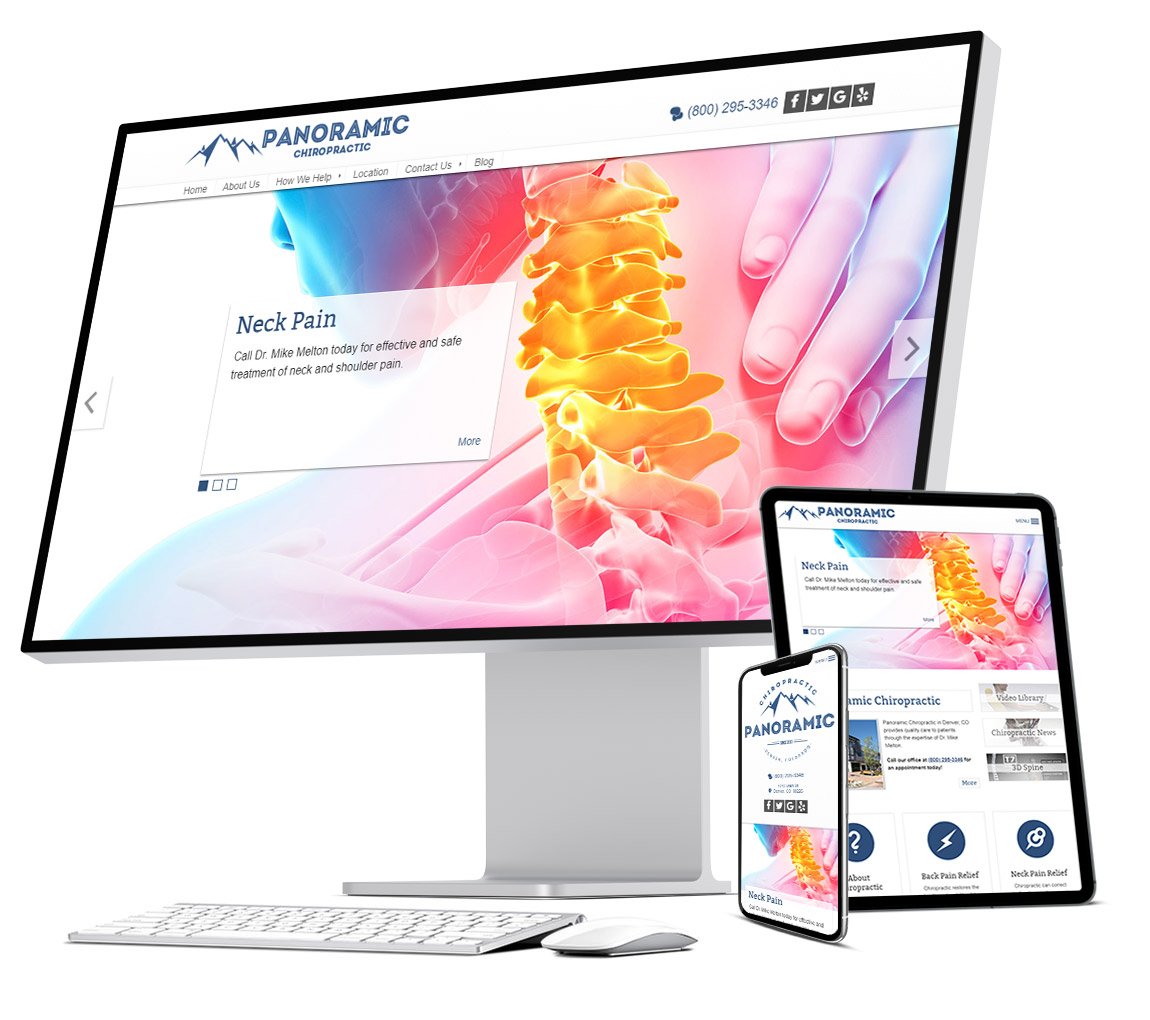
Grow with expert online marketing.
Amazing chiropractic online marketing plans, because you need to stand out above your competition.
Most Popular
Professional
Grow your practice while facing moderate competition